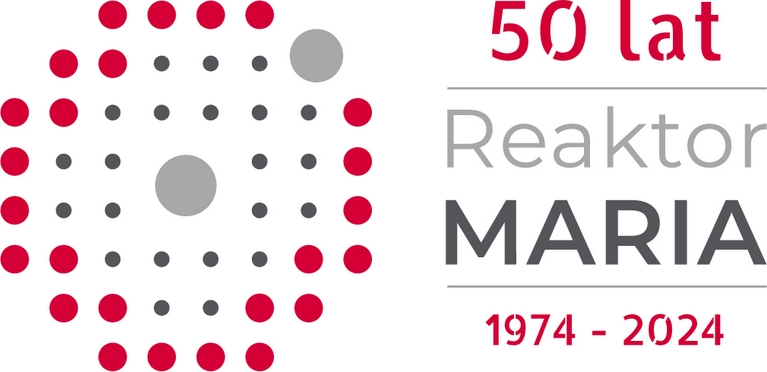
1. MARIA: What is it?
Poland's busiest MARIA is the country's only operational research nuclear reactor. It uses the neutrons created during a controlled nuclear fission reaction to produce radioisotopes and conduct research. The radioisotopes created in its core, which is the heart of every nuclear reactor, are used in medicine for diagnosis and treatment and in industry. With her work, MARIA is helping hundreds of thousands of people.
Despite being 50 years old, MARIA is one of the youngest and most modern nuclear research reactors in Europe and, thanks to modernisation, will be able to operate for many more years.
2. MARIA: Where is it?
MARIA, hidden under a distinctive round concrete dome and accompanied by fan chimneys (much smaller than those of typical nuclear power plants), is situated amidst the picturesque forests of Mazovia. It is the pride of the National Centre for Nuclear Research, one of the largest scientific institutes in Central Europe. MARIA is located at the NCBJ headquarters in Świerk, a district of Otwock. The Institute conducts both basic and applied research, mainly in the fields of physics, especially nuclear physics, but also materials engineering, electronics, astrophysics, environmental engineering, energy and pharmaceutical sciences. The MARIA reactor is an important facility for production and research related to most of these.
3. MARIA: What does it produce?
The main task of the MARIA reactor is the production of radioisotopes. There are dozens of isotope channels in the core of the reactor, into which the shield trays are placed. Depending on their location, neutron radiation of different intensities and energies acts on the target material. The disks spend at least several tens of hours in the MARIA core, during which time stable isotopes under the influence of neutrons become radioactive isotopes. The discs thus irradiated leave the reactor core and are processed, e.g. into radiopharmaceuticals.
The radioisotopes that MARIA produces the most are iodine-131, molybdenum-99, sulphur-35, phosphorus-32, samarium-153, holmium-166, ytterbium-169, cobalt-60 and lutetium-177.
4. MARIA: What do they put in her core?
In MARIA's core, mainly target materials are placed for the production of radioisotopes. These materials are placed in special aluminium irradiation trays, where they go into so-called isotope channels. These are specially prepared areas in the core where precisely known neutron radiation acts on the trays with the target material. In this way, scientists are able to determine precisely how long a particular shield must spend in the reactor core for the radioactive isotope produced in it to reach the desired activity. Once this has been achieved, the trays are transported to hot chambers shielded by a thick layer of lead and lead glass. Operations on the irradiated trays are performed with special manipulators, requiring great skill and precision from the operators.
In addition to production, the reactor core also receives materials for testing. These are exposed to the neutron flux to find out how the radiation affects the properties of the material. Because of the extremely high neutron flux that occurs at MARIA, a few weeks or months spent in the core corresponds to up to several decades of radiation in a power reactor. This allows scientists to test whether a particular material can withstand the predicted operating time in another reactor. Often such materials are additionally placed in special probes where they can reach much higher temperatures than in the MARIA core itself. Thus, in the reactor, the researchers reached a temperature of 1,000 degrees Celsius in the probe.
5. MARIA: Why does it shoot sideways?
In addition to the vertical channels through which containers of target material enter the MARIA core, there are also so-called horizontal channels in the reactor. Thanks to these, neutron beams with preset parameters can be derived from the core. Such a beam goes to the physics hall, where it can be directed to research equipment such as diffractometers and spectrometers. In this way, scientists are able to use the beams to study, among other things, the scattering of neutrons, thus obtaining information on the internal structure of materials.
A new stand for biological and medical research is being set up at one of the horizontal channels. The low-energy neutron beam coming out of the channel can be used in research into boron-neutron therapy, which will effectively fight certain types of cancer.
6. MARIA: What is it not suitable for?
Unlike nuclear power plants, in the MARIA reactor the heat generated during the fission reaction is purely waste. What is most important in a nuclear research reactor is the strong neutron flux with which other materials can be irradiated, not the energy production. In MARIA, the heat generated is dissipated through a pressurised fuel channel cooling circuit and a pool cooling circuit. Both of these circuits dissipate the heat into a common secondary circuit, which in turn is connected to a fan cooler where the heat goes into the atmosphere.
7. MARIA: How does a reactor work?
A nuclear reactor is a device that allows a controlled nuclear fission reaction to take place. The fissile material in the fuel, which in this case is uranium-235, breaks down into lighter elements under the influence of neutrons. In the process, a large amount of energy is released and further neutrons are released to trigger further fission reactions.
The neutrons produced during fission reactions have a very high energy, too high to easily cause further decays. To do this, it is necessary to take away some of their energy, i.e. slow them down. A moderator is used for this, the role of which is usually played by water. Neutrons collide with light atoms and, because of their similar mass, easily lose their energy.
To control the rate of the ongoing fission reaction, there are control rods and compensation rods in the reactor. Depending on how deep they are inserted into the core, they absorb excess neutrons and make the reaction occur stably.
The energy from the fission reaction, which is mostly the kinetic energy of the fission products, causes the fuel to heat up. This heat must be dissipated through the cooling system and can be (as in a power reactor) used to produce steam and further electricity, or (as in the case of a research reactor) removed from the core and discharged into the atmosphere.
8. MARIA: How is it built?
MARIA is a reactor with a rather unusual design, the so-called channel-basin reactor. The fuel channels, which contain specially prepared elements containing fissile uranium, together with beryllium blocks (which are the moderator) and graphite blocks (which are the reflector - they return the neutrons that escape from the core) form the core of the nuclear reactor. This core is submerged in the reactor pool under a seven-metre layer of water. Also part of the core are the isotope channels, into which the storage tanks of irradiation material go, as well as the absorber rods, compensation rods and safety rods to control the fission reaction.
The reactor pool is surrounded by a steel liner and a thick layer of concrete which, together with the water filling the reactor pool, provides a biological shield. The reactor pool is also connected by a sluice to the adjacent storage pool, which receives spent fuel elements and irradiated structural components.
The core in the MARIA reactor is modular and can be adapted to a specific production or research programme to achieve the necessary parameters, especially neutron flux and energy.
9. MARIA: Will it not explode?
MARIA's safe operation is guaranteed by a number of safety systems. The control, safety and process control systems ensure that all reactor controls are operating properly, and collect data on the fission reactions taking place, as well as other physical and technological processes occurring during normal reactor operation. The primary and secondary systems are responsible for cooling the reactor fuel elements. These systems, which receive the heat from the fission reaction during reactor operation and the post-shutdown heat after reactor shutdown, are equipped with additional pumps that are able to cool the reactor even in the event of an accident. An additional safety system is also responsible for shutting down the reactor in the event of an accident. The system blocks the start-up and ramp-up of the reactor and, as a last resort, causes emergency flooding of the core and extinguishment of the fission reaction.
Although MARIA does not produce electricity itself, it requires it to operate properly. The power systems that supply electricity to all the necessary components are also adequately protected - MARIA is powered by three independent power lines, ensuring continuous and safe operation under both normal and emergency conditions. There is also a dosimetry system in the reactor building, which is responsible for monitoring radiation in the reactor building, as well as for radiological protection of personnel. In addition, it includes an assessment of the risk to the population resulting from the operation of the MARIA reactor.
10. MARIA:Does it glow and produce waste?
In many photos and videos showing MARIA, the characteristic blue light emanating from its core is visible. This is not the result of lamps installed in the reactor pool, but a natural physical phenomenon - Cherenkov radiation. The nuclear reactions occurring in the reactor core produce charged particles with high energies. These particles enter the water surrounding the core and cause polarisation of the atoms close to which they fly. As they return to their previous state, the atoms emit some of their energy, visible as a characteristic blue light.
Nuclear fission reactions consume fuel - uranium-235 decays into lighter elements that remain in the fuel elements. However, even after the reactor is shut down, nuclear reactions continue to take place in the fuel, e.g. from decaying radioactive isotopes. Spent nuclear fuel is stored and cooled in a pool next to the reactor pool for at least a few years, after which it is transported to a manufacturer for further reprocessing.
In addition to spent fuel, the operation of the MARIA reactor is associated with the generation of other wastes. All of these, whether in solid, liquid or gaseous form, are carefully monitored.
Low- and intermediate-level solid and liquid wastes go to the nearby Radioactive Waste Disposal Facility, which processes and prepares them for disposal at the National Radioactive Waste Repository in Różan. The gaseous waste passes through a ventilation system where it is filtered. Carefully controlled quantities of certain gases, mainly argon-41, formed by air activation, are released from the reactor stack during normal operation.
11. MARIA - history: Where did it come from?
Just less than a decade after the first EWA nuclear reactor in Poland became operational, a plan was drawn up in 1964 to use a second research reactor. Like its predecessor, the new reactor was to be used to irradiate targets for the production of radioisotopes, but there was also a strong emphasis on scientific research. The new design was to enable physical, radiochemical, materials research in special probes and loops, as well as materials engineering and nuclear power research. Two years later, the decision was taken to build the MARIA reactor, named, of course, after the two-time Polish Nobel laureate, Maria Skłodowska-Curie.
Unlike EWY, which was a Soviet-designed reactor, MARIA was designed and built entirely by Polish specialists and technicians. Construction began in 1970 (the ceremonial laying of the foundation stone into the building's slab took place on 16 June), and the reactor achieved criticality for the first time on 18 December 1974 at 0:17.
12. MARIA - history: Did she have older sisters?
The first research nuclear reactor built in Poland was the EWA reactor (the name is an acronym for Experimental, Water, Atomic). The reactor was purchased from the Soviet Union and commissioned at the then Nuclear Research Institute in 1958. EWA was a WWR-S type reactor with a thermal power of 2 MW. Polish scientists made many changes, increasing the reactor's power fivefold, to 10 MW and periodically up to 12 thermal MW. EWA primarily produced radioisotopes for medicine and industry and operated without any failures for 37 years. The reactor was shut down in 1995 and its decommissioning was completed in 2002.
Several so-called zero-power reactors were also built at the Institute (they are called rak reactors with a real power of the order of 100 W, which do not require active heat dissipation). The first one built at Spruce was the ANNA critical set, which was built in 1961-63, designed and built entirely by our specialists. ANNA was intended for scientific research work and, for a time, the production of radioisotopes. In 1972, ANNA underwent a major modification that allowed it to operate on natural uranium without a moderator. This design uses fast neutrons for fission (where in MARIA the reactions are caused by slowed neutrons, so-called thermal neutrons), hence the set was renamed P-ANNA (Fast ANNA).
Another zero power reactor was MARYLA, commissioned in 1963. MARYLA was used for research into the possibilities of operating the EWA reactor at variable and increased power, as well as research into the use of a different fuel type. The MARYLA assembly operated in a number of configurations, designated, for example, as MARYLA-1 and MARYLA-2, and approximately 180 different configurations were tested during the research. The MARYLA reactor ceased operation in the mid-1970s. Based on the last modifications of the MARYLA reactor, the 100kW UR-100 university reactor, also called the WANDA reactor (Water, Academic, Scientific, Teaching, Application), was to be developed. The UR-100 was designed with the possibility of series production for universities in mind. The reactor underwent successful criticality tests at Świerk, after which the prototype was transported in 1985 to the AGH University of Science and Technology in Kraków, where, however, it was never commissioned.
The HELENA sub-critical set was also developed in Świerk (the sub-critical set does not reach the critical mass needed for the fission chain reaction and needs an external neutron source). The set was built in 1963 and was used to study the physical parameters when operating on natural uranium fuel. The HELENA set was dismantled around 1980.
The last critical assembly to be built before the MARIA reactor was the AGATA reactor, commissioned in 1973. This was a zero power reactor of very similar design to MARIA and initially tested the fuel on which MARIA was to operate. AGATA also served as a training reactor for future MARIA operators, and mock-ups of water loops were tested there before being used in the MARIA reactor. AGATA ceased operation in the 1980s.
13. MARIA - the future: will it live to be 100 years old?
Since its start-up in 1974, MARIA has undergone a number of upgrades during which the systems operating in the reactor were replaced and improved. The first major upgrade took place in 1985, during which the reactor control system, cooling system equipment, air conditioning and ventilation, among others, were replaced. In the 1980s, as a result of the Chernobyl power reactor accident, MARIA gained new safety systems such as the fuel channel flooding system. The biggest changes to the MARIA reactor were the transition from highly enriched (80% fissile uranium-235 content) to medium and then low-enriched fuel (less than 20% 235U content). The conversion process to low-enriched fuel lasted from 2000 to 2014 and was combined with an upgrade of the cooling circuit.
The most recent series of upgrades was completed in 2023 and involved the main switchgear and control rooms of the reactor, as well as parts of the measurement systems and the fan cooler. Although MARIA already has half a century on its shoulders, thanks to continuous improvements it is still one of the most modern research reactors and will be able to operate until at least the 1950s of the 21st century.
14. MARIA – the future: will she have a sister?
Soon, MARIA may no longer be the only nuclear reactor in Poland, but it is possible that there will be a sister at Świerk. A high-temperature gas-cooled reactor (HTGR) project is being prepared at the NCBJ. This reactor is designed for industry, as it allows the production of high-temperature steam (with temperatures in the order of several hundred degrees Celsius), which is necessary for some industrial processes. The HTGR reactor also includes the possibility of so-called cogeneration, i.e. the production of both heat and electricity. A scaled-down version of the reactor (30 MW of power versus 160 MW of the industrial variant) called HTGR-POLA is to be built in Świerk. It will serve as a demonstrator of this technology in Poland prior to the launch of the industrially directed variant.
In addition, although MARIA will not be retired for many years yet, plans are already emerging for its successor, which will take over radioisotope production duties and allow it to continue to provide medicines to hundreds of thousands of patients, as well as conducting new physical research.
15. MARIA – the future: the power station? How can we help you?
NCBJ is authorised by the State Atomic Energy Agency to support the President of the PAA in assessing radiological consequences resulting from safety analyses carried out for various states of nuclear power plants, probabilistic safety analyses of nuclear power plants and nuclear physics issues in practical applications in nuclear power plants. This is the first step for NCBJ to apply for the role of Technical Support Organisation (TSO) for the PAA in the process of licensing and supervising the construction of nuclear power plants in Poland.
A Technical Support Organisation (TSO) is an institution that aims to provide technical support to the nuclear sector, including power plants and other nuclear installations, as well as oversight institutions. TSOs must meet requirements for independence, technical expertise, access to information, compliance with regulations and standards, and training and professional development.
16. MARIA – the future: do we want students here?
MARIA, as a unique research reactor, requires many specialists to operate it. These are not just reactor operators, but also technicians who are responsible for the various electrical, hydraulic and other systems. Half a century of MARIA's operation means that generational exchanges must take place in order to maintain knowledge and competence in the operation of the reactor's systems, as well as the research carried out on reactor physics. At the NCBJ, for example, competence has been rebuilt in the design and conduct of research in the high-temperature probes that were originally placed in the reactor in the 1970s.
For this reason, training courses and workshops are organised at NCBJ, including those conducted at the MARIA reactor. These are aimed especially at students who want to start a career in nuclear physics and energy and allow them to become familiar with reactor measurements or reactor safety. Participants have the opportunity to take part in a reactor start-up and an operator working day, as well as learning about the design process of the irradiation bins and the preparation of the materials themselves before being placed in the core.
17. MARIA at NCBJ: Where do the radioisotopes go?
The material irradiated in the MARIA reactor cannot be used directly. The desired isotope is among many others in the shield and needs to be separated and processed to become useful. By virtue of the fact that the shield material has spent many hours in the MARIA core, it becomes highly radioactive and work on it must be carried out in a special way. From the reactor, such material goes into so-called hot chambers. These are special structures surrounded by a thick layer of lead to protect the operator from radiation. The view inside the chamber is also shielded - it takes place through a layer of lead glass several tens of centimetres thick. The operations on the irradiated containers themselves are carried out by the operators using special, high-precision manipulators, which enable them to prepare such material for further transport. In the chambers, the irradiated material is transferred to containers, also made of a thick layer of lead, in which it is transported for further processing. In this form, radioisotopes are sent, for example, to POLATOM, where in special installations, also located in hot chambers, the desired isotopes are extracted from the target material and processed for further processing. Ultimately, the radioisotope produced in the MARIA reactor, for example, takes the form of a drug - a radiopharmaceutical that can be administered to a patient to diagnose or treat cancerous lesions.
18. MARIA at the NCBJ: Why is CERAD needed?
The MARIA reactor is a unique device that can produce a great many radioactive isotopes. However, our MARIA also has its limitations. Materials are irradiated by interacting with a very intense neutron beam. Unfortunately, not all isotopes can be produced by interacting atoms with neutrons. For this reason, among others, the CERAD project - Centre for the Design and Synthesis of Molecularly Targeted Radiopharmaceuticals - is being implemented at NCBJ. A key element of the project is the cyclotron, a type of accelerator. This device allows the production of beams of charged particles - protons, deuterons (deuterium is an isotope of hydrogen with one proton and one neutron in the nucleus) and alpha particles (an alpha particle is a helium nucleus - 2 protons and 2 neutrons). Such beams can be bombarded with other target materials so that we can produce other radioisotopes. In this way, scientists at the POLATOM Radioisotope Centre will be able to design new radiopharmaceuticals for the treatment of specific types of cancer. This will also be facilitated by modern laboratories with the necessary infrastructure, which are also part of the CERAD project.
19. MARIA at NCBJ: How to design a new drug?
In radiopharmaceuticals, two elements are most important - the radioisotope and the biological molecule to which it is attached. The radioisotope emits radiation that can destroy cancer cells or be detected by tomographs to locate lesions. Depending on the intended use, the radioactive isotopes that are to become part of the radiopharmaceutical are selected according to their properties.
The most important of these are the half-life and the type and energy of the emitted radiation. The half-life determines how long the radioisotope will be active in the patient's body before it decays to a stable form. This value is crucial, as it can be neither too short nor too long. A decay time that is too short, e.g. of the order of a few tens of minutes, will result in most of the isotope disappearing even before it gets from the reactor to the laboratory, where it will take the form of a drug. Add to this the time it takes to transport the finished drug to the patient, and he or she would have taken in only a small fraction of the radioisotope that left the MARIA core. Conversely, excessively long decay times, on the order of days or weeks, mean that the isotope would emit radiation in the patient's body all the time, from administration, through to reaching the target tumour area, and further on, when leaving the patient's body. This, in turn, carries a higher risk of irradiating healthy cells and further complications related to radioactive waste disposal. Therefore, the preferred half-life values are of the order of a few to tens of hours. Such isotopes can be processed and transported before most of them have disappeared, and they decay rapidly in the patient's body, after which they can be safely disposed of.
The second extremely important factor is the type and energy of radiation emitted. Depending on how an isotope decays (whether alpha, beta or gamma decay), it will be for different purposes. Alpha decay usually means the emission of a particle of fairly high energy, but with a very short range, on the order of millimetres. Such a radioisotope can go directly to the tumour site, where the alpha particles will destroy the cancer cells. Beta particles already have a longer range and are therefore used in other cases. Gamma quantum emissions, on the other hand, can be used for diagnostics to detect clusters where this isotope accumulates.
The thirs essential element of a radiopharmaceutical is the biological molecule. This must be specially selected so that the radioisotope in question can be attached to it. In addition, it is chosen so that it readily accumulates in the target area, such as a particular organ where cancer cells may be present.
However, finding a matching combination of radioisotope and molecule does not yet mean that a radiopharmaceutical is marketed. Before that, lengthy studies must be conducted to ensure its efficacy and safety. Only after undergoing rigorous research and testing can a new radiopharmaceutical be licensed and serve patients.
20. MARIA at NCBJ: How and why is a new material designed?
With advances in science, engineers are able to design and manufacture specialised materials to achieve precisely defined properties. Modern metal alloys, ceramic and other materials are designed for this purpose. Specific material properties are sometimes necessary to make components that work in the target, sometimes extreme, environment.
Such an environment can be, for example, exposure to ionising radiation, which is the case for materials used in the construction of nuclear installations such as power plants. The materials used in such constructions have to withstand decades in the continuous vicinity of radioactive fuel and the safety of the entire installation depends on them. It is therefore very important to design and thoroughly test the materials to be used for such purposes. Fortunately, this is also where the MARIA reactor can come to the rescue. The neutron radiation that occurs in a research reactor such as MARIA has a unique property - it is very penetrating. Therefore, by placing some material in its field, it only takes a few weeks or months to get the same level of wear that would occur in an actual nuclear power plant after many years.
In the MARIA reactor, such materials can additionally be placed in special high-temperature probes which, as the name suggests, in addition to strong radiation, expose the material to prolonged high temperatures (of the order of several hundred or even a thousand degrees Celsius) to further reflect the conditions under which the material must operate for several decades.
21. MARIA at the NCBJ: count, count and count?
Although the MARIA reactor core is itself a fairly small structure, for proper operation and irradiation of materials, operators need to know exactly the conditions inside. Additionally, due to the structure of the core and the possibility of creating different configurations of its elements, parameters such as the neutron flux at a given location or the heating rate of the fuel elements can vary dramatically for different configurations. For this reason, thermal-flux scientists play a very important role in the MARIA reactor team. These are physicists who, with their knowledge of the arrangement of the elements in the core and their vast knowledge of reactor physics, are able to accurately determine the conditions in the MARIA core. With the help of special programmes, they can model the distribution of heat generated in the fuel elements and also predict how strong the neutron radiation acting on the target material will be. This makes it possible to plan exactly how long such material needs to spend in the core in order for the radioisotope formed in it to reach the desired activity and become useful for reprocessing.
Depending on the needs, this type of calculation can use different ways of modelling the reactor interior and its fuel elements. Computational fluid dynamics, which uses numerical methods to solve fluid flow issues, is often used for this. Unfortunately, obtaining accurate results in this way requires very high computing power. Our scientists can use the NCBJ's Computer Centre Spruce, which has a powerful supercomputer at its disposal, for this purpose.
22. MARIA at NCBJ: What is worth measuring and how?
The intense neutron flux that is generated in the MARIA reactor can, thanks to the presence of horizontal channels, be routed out into the physics hall, where it reaches special research equipment. One type of such equipment is diffractometers, in which a beam of radiation (usually X-rays, but in this case a neutron beam from the reactor) falls on the material under study and is deflected. From the image thus obtained, scientists are able to gain information about the material. In this way, it is possible to study, for example, the inhomogeneities present within the material, as well as the quality of the metal monocrystals produced. Another type of instrument that can make use of a neutron beam is a spectrometer, which makes it possible to study, for example, the collective movements of atoms or the energy levels of ions in a solid. This type of information can be obtained by inelastic neutron scattering.
Neutrons from the MARIA reactor can also be used in radiographic studies. These take advantage of the penetrability of neutron radiation so that the internal structure of objects that are opaque to visible light can be seen accurately. In this way, researchers can learn exactly about the internal structure of technical devices. Furthermore, it is possible to observe the movement of the object being x-rayed, so-called dynamic radiography (e.g. observing the operation of a moving piston). The use of radiography also allows other processes to be studied, such as the migration of water in porous materials or the development of the plant root system.
An interesting use of radiography is also the study of paintings, the so-called neutron autoradiography. By irradiating the painting with low-energy neutrons, radioactive isotopes such as phosphorus-32 are produced in the layers of the applied paint. This isotope then decays, which can be recorded on X-ray film. In this way, an image can be obtained on the film that corresponds to the individual pigments, including those found in the deeper layers of the paint. In this way, researchers learn that there are other paintings hiding beneath some of the paintings.
23. MARIA at NCBJ: Can we train you?
NCBJ's Education and Training Department (DEiS) has, for more than 20 years, been involved in, among other things, organising training courses on various topics, primarily in nuclear physics. The nuclear industry requires a great deal of knowledge and competence in the field of nuclear technology and radiation safety, so professional education and specialised training are extremely important. The training classes organised by the DEiS team provide practical skills by using the Institute's research infrastructure. This form ensures that a new generation of nuclear professionals will develop this industry and keep it safe.
24. MARIA at NCBJ: Do you want to see and understand?
The MARIA reactor is a unique facility on a national scale. Although it fulfils a very important role and is closely monitored in terms of safety, especially radiological safety, it is not a facility cut off from the world. The NCBJ Education and Training Department offers tours of the Institute for organised groups, primarily school groups. Tours include visits to laboratories as well as the MARIA reactor (due to the situation still prevailing, tours of the reactor have been temporarily suspended), combined with lectures during which participants learn details of their work.
A number of multimedia materials are also available on the MARIA reactor, as well as other NCBJ departments and facilities, including the NCBJ and OJ Spruce's Virtual Open Days video series and a dedicated video series on the MARIA reactor. All these videos, as well as many others, including popular science lectures, can be found on NCBJ's YouTube channel. You are cordially invited to watch them and learn new facts about the life of Poland's busiest MARIA!
25. MARIA in numbers: What does it look like?
Origin of the plan to use the MARIA research reactor: 1964.
Start of construction: 16 June 1970.
MARIA reaches criticality for the first time: 18 December 1974, 0:17 a.m.
Nominal thermal power: 30 MW
Maximum thermal neutron flux density: 3.5*1018 n/(m2*s)
Annual operating time: 4000-5000 hours
Irradiation cycle time: 20-300 hours
Number of fuel elements in the core: 18-26
Maximum power generated from a fuel element: 2 MW
Maximum energy generated from a fuel element: 6000 MWh
Uranium-235 fuel content: 19,75%
Amount of uranium-235 in fuel element: 485 g
Size of fuel element: 1 m
Number of isotope channels: 22
Maximum number of irradiated pools in the core: 198 per cycle, 4500 per year
Reactor pool depth: 10,45 m
Thickness of concrete cover of reactor core: 220 cm
Reactor core size: 1,5 m in height, 2,25 m in width
Size of reactor pool: bottom cylinder - 4,5 m in diameter, 5,1 m in height; top part floor plan being semicircle and trapeze - 3,25 m in radius, 5,35 m in height
Size of storage pool: 12,35 m x 3,5 m, 6,5 m in height
Vent stack height: 60 m
Water pressure in the cooling circuit: 1.7 MPa
Cooling circuit water temperature : 50 °C (inlet), up to 80 °C (outlet)
Reactor pool water temperature: up to 45 °C
Maximum fuel element sheath temperature: 155 °C
Number of operators in the reactor control room: 2
Number of technicians working on the reactor: 5 per shift, 50 in direct operation, 100 in total